Using stroke rehab equipment at home often motivates survivors to exercise on a regular basis. Exercising daily helps keep the brain stimulated and encourages neuroplasticity, the mechanism the brain uses to rewire itself, which is key during stroke recovery.
Written exercises alone can help, but adding useful stroke rehab equipment expands the type of exercises you can do. To help you find the right gear, this post provides a roundup of the best stroke rehab equipment available for you to use at home.
All Amazon links are non-affiliate, which means we do not receive any compensation when you click on them. The products from Flint Rehab are our own. Let’s get to it!
Use the following links to jump directly to a section:
How to Choose the Right Equipment for You
Stroke Rehab Devices That Help Stroke Survivors Walk
Premium Stroke Rehab Equipment
Arm and Hand Stroke Rehab Equipment
Stroke Rehabilitation Equipment for Aphasia
How to Choose the Right Equipment for You
As you shop around, it’s important to look for tools that you find motivating. After all, results are created through repetition and consistency. Look for devices that you can see yourself using it every day.
Activating neuroplasticity through repetition and consistent therapy is how you will see lasting long-term results.
While all stroke rehab equipment aims to improve your function and mobility, not all strokes are equal. In fact, every stroke is different, which means that every recovery will be different.
Not every stroke survivor will need everything on this list. In fact, there may only be a few items that target your unique needs. It’s important to be clear on your recovery goals so that you can choose the right equipment.
If you feel lost, your therapist is a great resource. You can also look for recovery tools that are similar to the ones you used during your time with your therapist.
To help you find even more clarity, consider these questions as you look for the best stroke rehab equipment for your goals and needs:
- Does this help me achieve high repetition?
- Can I see myself using this consistently?
- Does this make me excited to do therapy?
If something ticks all these boxes, it will make a great investment on the road to recovery.
Stroke Rehab Devices That Help Stroke Survivors Walk
Almost 80% of stroke survivors struggle with hemiparesis, which involves weakness on one side of the body. When this occurs, survivors are more prone to balance difficulties and issues with their manner of walking (gait).
Some of the best devices that help stroke survivors walk again are those that target the feet, legs, core, and arm. These major muscle groups all contribute to your gait. It’s a full-body task!
If you want to improve your ability to walk, then consider these gadgets for stroke survivors that target the legs, core, and possibly more:
Stationary Recumbent Bikes
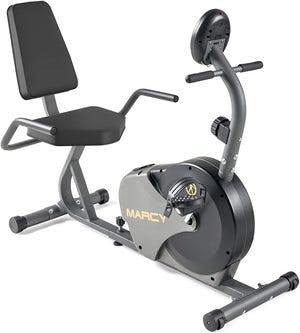
Stationary recumbent bikes are an excellent tool for stroke survivors that want to build up the skills necessary for walking. Bikes encourage bilateral movement, which is helpful for individuals with hemiparesis because the non-affected leg can assist the affected leg. Although this may feel like cheating, it’s actually helpful for rehabilitation because it stimulates the brain.
Unlike regular stationary bikes, recumbent bikes are often a better fit for stroke survivors because they offer more support and a gentler experience.
Shop recumbent bikes on Amazon »
FitMi Full-Body Home Therapy
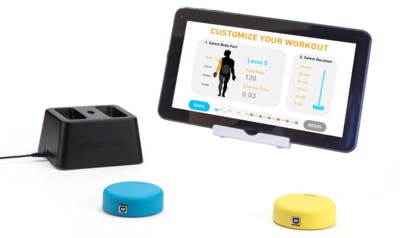
The FitMi is an award winning home rehab program that helps increase hand, arm, core, and leg function after a stroke or other neurological injury. It is interactive and allows you to play along in a game like structure which helps motivate you to exercise your full-body.
When you start your exercise session, you get to choose which muscle groups you want to target: legs, core, arm, and/or hand. Then, FitMi selects appropriate rehab exercises based on your ability level.
FitMi has helped many survivors regain the ability to walk and accomplish other daily activities, like driving and typing. The device tracks your progress and “levels up” your exercises when you’re ready to keep you optimally challenged. It’s a great way to stay engaged in therapy at home between visits with your therapist.
The FitMi is clinically proven to improve mobility 3x faster than traditional therapy alone with 20 minutes per day.
Balance Boards
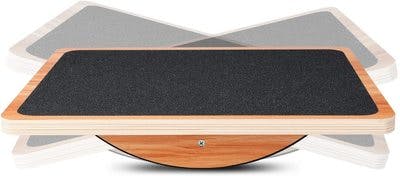
When you stand on a balance board — making sure to hold onto a table for stability when first starting out — it helps challenge your balance and target your vestibular system. These are essentials for survivors that want to improve their ability to walk.
Balance boards with rocking bases, like the one shown above, offer more stability than balance boards with a single point. Work your way up from one to the other, and always be safe by using these tools with caution.
Shop balance boards on Amazon »
Stability Balls
A stability ball is a simple piece of stroke rehab equipment, and it’s safer to use than a balance board. It’s commonly used for core rehab exercise; and a strong core is essential for improving your balance and ability to walk.
Shop stability balls on Amazon »
Dumbbells and Wrist/Ankle Weights
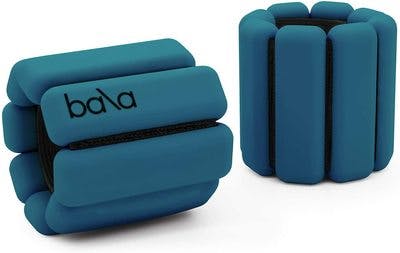
Stroke recovery isn’t all about range-of-motion and mobility, though. Strength training is important for addressing any muscle atrophy that may have occurred after long periods of inactivity.
To increase strength in your arms and legs, dumbbells and wrist weights are an affordable, simple option. Use them to complete upper extremity exercises to not only stimulate the brain and encourage recovery, but to stimulate muscle growth too. You can often attach wrist weights to your ankles to get double the use from them, too.
Shop adjustable wrist weights on Amazon »
Premium Stroke Rehab Equipment
Not all stroke survivors need premium equipment in order to see results. However, premium stroke rehab gadgets can be motivating to use, which helps encourage consistent therapy.
Since repetition and consistency are key to recovery, consider these premium stroke recovery tools to use at home. It’s always a good idea to run them by your therapist to ensure they’re a good fit for you.
MusicGlove Hand Therapy
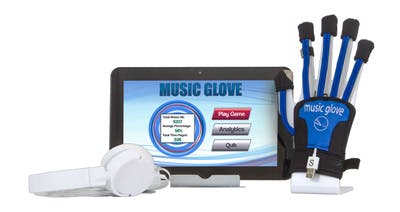
MusicGlove is a music-based hand therapy device that’s clinically proven to improve hand function within 2 weeks when used for 25 minutes a day. It works by motivating you to practice hand therapy exercises while playing along to a musical game. During a single session, most users accomplish hundreds of repetitions, which helps stimulate the brain and promote neuroplasticity.
If you’re looking for something to motivate you to exercise your hand every day, MusicGlove is the perfect fit. It also makes a great gift for stroke survivors because it’s fun to use and some survivors even forget they’re doing therapy while they use it.
Shop MusicGlove from Flint Rehab »
Arm Peddlers
Arm peddlers provide another form of bilateral exercise. This means that both your arms are moving at the same time. Sometimes the under-desk stationary bikes can be used as arm and leg peddlers, if you’re looking for a creative way to get double the use out of your stroke rehab equipment.
Electrical Stimulation Machine
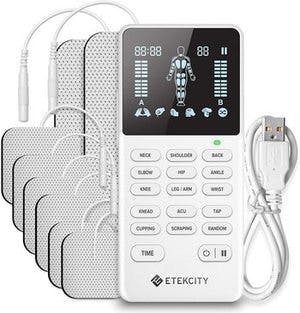
Electrical stimulation is an effective, evidence-based tool for stroke survivors, especially those with paralysis on one side the body (hemiplegia) or severe muscle weakness. It works by attaching pads to the skin over the desired muscles and sending gentle electrical stimulation, which helps the muscles contract. It’s a great way to introduce movement to paralyzed muscles and stimulate the brain.
Before getting started, it’s important to work closely with your therapist to learn where to place the pads and how to safely operate the equipment. The placement is not always intuitive, so it’s essential to receive guidance from your therapist.
Shop electrical stimulation devices on Amazon »
Arm and Hand Stroke Rehab Equipment
After targeting larger muscle groups, it’s important to target the hand and fingers. Fine motor skills are essential for accomplishing the activities of daily living such as brushing your teeth or using utensils, and there are many stroke rehab devices that can help with these goals.
To improve arm and hand mobility, consider these useful stroke recovery tools:
Mirror Box
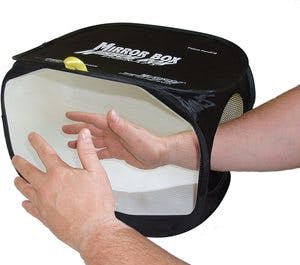
Mirror therapy is a hand rehabilitation method that involves using a tabletop mirror to create a reflection of your non-affected hand. When you exercise your non-affected hand, it “tricks” your brain into thinking that both hands are moving, which helps spark neuroplasticity and encourage movement in the affected hand (even though you’re not intentionally moving it).
If you struggle with hand paralysis or severe spasticity (like a clenched hand), mirror therapy is a great option.
Arm Skates
At first glance, arm skates may look like boring stroke recovery tools, but they can be helpful for increasing range-of-motion by limiting the amount of work required. They are not used for strength training. Rather, they help reintroduce movement to the affected arm.
Therapy Putty
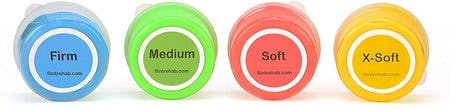
Therapy putty is popular physical therapy tool that can help improve fine motor coordination, strength, and range of motion. Some hand strengthening exercises involve squeezing the putty while some coordination exercises include finger extension. We cover all these exercises in our therapy putty exercise guide.
Shop therapy putty on Amazon »
Hand Exercise Balls with Extension Add-On
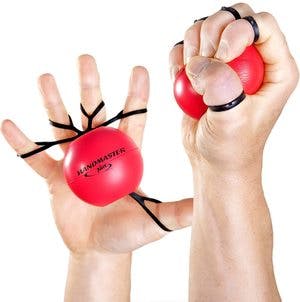
Typical hand exercise balls look like average stress balls, which can be a helpful tool during stroke recovery. For a slight upgrade, you can try these exercise balls that also come with finger loops for finger extension, too. This helps you target both flexion and extension without skipping a beat.
Shop hand exercise balls on Amazon »
Stroke Rehabilitation Equipment for Aphasia
Not all stroke rehab equipment is designed to help with movement. There are tools that help with communication and cognition, too.
These tools are great for individuals with aphasia, a communication disorder, or other cognitive difficulties like poor concentration and memory. Just like practicing rehab exercises helps improve movement, practicing cognitive exercises helps improve cognition.
Here’s our recommended too for improving speech, communication, and cognitive skills after stroke:
CT Speech & Cognitive Therapy App
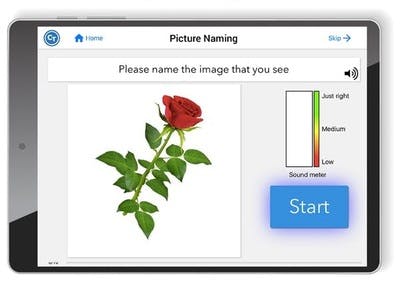
The CT Speech & Cognitive Therapy App provides over 500,000+ speech and cognitive exercises to help people recovering from stroke, brain injury (TBI), or those living with aphasia, apraxia, dementia, and more.
When first getting started, the app assesses your needs and ability level and then assigns the appropriate exercises for you to practice. It’s like having a virtual speech therapist available any time you want. And your own speech therapist can even help assign your exercises through the app!
The app has won multiple-awards from Hearst Health, UCSF Health Hub, Fierce Innovation Awards, the American Stroke Association, and AARP. It works on any Apple iOS or Android phone!
Shop the CT App from Flint Rehab »
It’s Time to Shop!
We hope this roundup of stroke rehab equipment helped you find the right tools for your recovery. Whether you go big with a recumbent bike and e-stim, or whether you go small with some simple therapy putty, they will all help if you use them regularly. Of course, we also hope there’s some fun in your future with Flint Rehab’s gamified neurorehabilitation devices.
Whatever you choose, we hope it makes you excited to do therapy. That’s how you will see the best results.